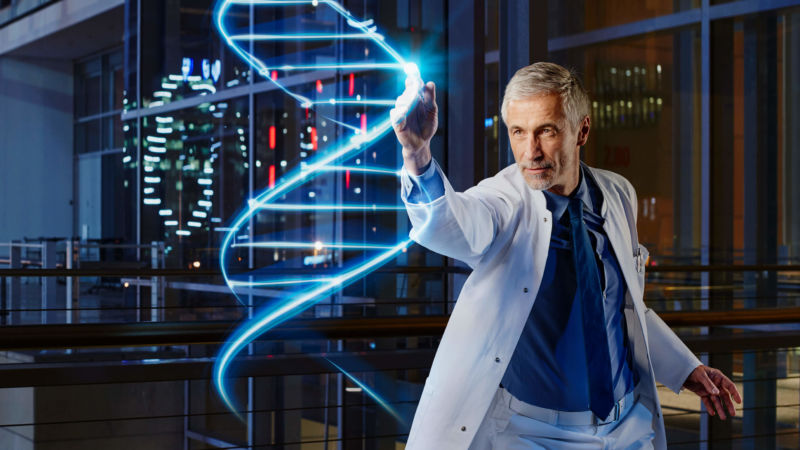
Roger Richter / Getty
Since the start of the millennium, we've improved the cost and quality of reading DNA 10 millionfold. This technology applies identically to our own genomes and to those of the most deadly pathogens. Yet we've barely begun to use this new “superpower” of DNA scrutiny to monitor our environment for threats to human health.
Many of the enabling technologies for highly distributed DNA detection networks are already here. For instance, we now have palm-sized devices that read DNA in nearly real time, and they can be attached to our smartphones—which themselves can append and transmit audio, video, and GPS data. Thousands are already using these new tools. They're based on nanopore and other single-molecule electronics—which have very low reagent and tiny fabrication costs, and they are super-portable (a fraction the size of a phone).
Like all things in synthetic biology, these tools will continue to improve, cheapen, and miniaturize with extraordinary speed. Future generations of sequencers could be widely distributed throughout our homes, offices, the broader biosphere, and even within our bodies. Deeply networked, they could alert us to pathogens in the next room or in distant parts of the world. A network like this would revolutionize epidemiology, potentially moving it from 10-year studies to 10-minute assessments.
Modern problems require modern solutions
Our ancestors were accustomed to spending their entire lives in walking distance of their birthplace, but our modern world is so 'well-stirred' that new biological threats could spread from anywhere on the globe to anywhere else in months, or possibly even days. Fortunately, information can spread even more rapidly. A global threat-detection network could help deliver on the huge promise this presents to public health. For instance, it could give us baseline measurements of variations in drug resistance as a function of seasons, droughts, floods, fires, construction, and more. This would let us detect new pathogen strains long before they spread, possibly even at their 'patient zero' point of origin.
Much like we’d check the weather in the morning before setting out on our commute, we might one day also check the pathogen forecast. Should we go to work—or our send our kids to daycare—given the profile of sniffles or cough afflicting everyone in the mix today? In answering this, should these detections depend on simple generic rules? Or on “precision medicine” knowledge of everyone's vaccination/exposure history, natural genetic resistance, and known drug resistance? And how should we respond to a newly detected pathogen—based on its transmissibility, novelty, evidence of genetic engineering, weaponization, or other tampering? Should we encourage researchers to sign strains on which they work using otherwise-neutral DNA marks or leverage natural marks, isotopes, and associated matter?
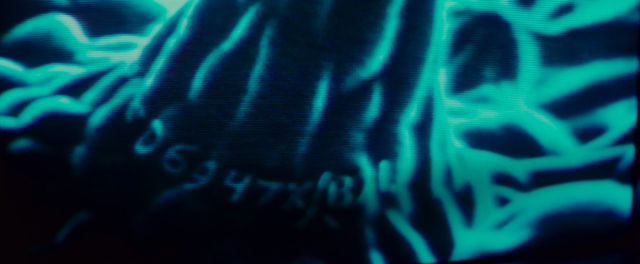
Warner Bros.
We won’t know the answers to these questions until we actually get to the process of laying out the full requirements of the detection network—and answering those questions is critical.
Inspector (DNA) Detector
To make a DNA-sensitive threat-detection network viable, we’d first need a more equitable distribution of medical technologies across the world, especially when it comes to DNA surveillance. Three of the most low-cost and highly effective global public health strategies are hygiene, quarantine, and vaccines—all of which require constant, keen observation to be effective.
In addition to tracking known diseases, we'll of course want to discover the next pathogen long before it’s an epidemic. Some ancient human scourges have proven amenable to extinction—for example, smallpox, polio, and guinea worm—and the hope would be that new threats would prove, after analysis, to be equally amenable. We could track a new threat’s progression in birds or pigs and monitor their drug resistance. We could track our own immune responses, including unwelcome autoimmune and allergic reactions, to a threat. Steve Elledge's lab (with a little early help from my lab) has shown that we could analyze immune response to all human proteins and all viruses with a fraction of a drop of body fluid.
We could start connecting environmental exposures and individual immune reactions to disease. Many diseases (including arthritis and diabetes) seem to involve interactions between the environment and immune systems. A networked biomonitoring system could both help establish or disprove that link and also potentially help us get much better at preventing these diseases.
Much like we’d check the weather in the morning before setting out on our commute, we might one day also check the pathogen forecast.
We're already moving toward minimally invasive, body-internal recording devices. Reza Kalhor in my group has recently recorded the whole lineage of development in mice, from egg to adult—a terabyte of information stored in only one billionth of the body mass.
A call to action
"If you see something, say something" is an oft-repeated maxim for public safety. A culture of awareness should also extend to scientific teams that might knowingly or unwittingly create artificial biothreats. Controversies have arisen the wake of 2001's killer mousepox modification, 2005's de-extinction of the 1918 H1N1-flu, 2011's "gain-of-function" modifications of the H5N1-flu virus, 2018's CRISPR babies, and many other instances. In each of these cases, non-participants are known to have had prior knowledge of the risky research,yet did not call for scrutiny. Potential whistleblowers should be incentivized by carrots and/or sticks.
Broad surveillance inherently opens up issues of civil liberties and invasion of privacy, but those issues must be properly contextualized—especially when it comes to the potential public health benefits. One potential precedent is our regulation of driving—which includes licensing, mandatory bans of recklessness and drunkenness, and surveillance tools like speed traps.
It is not our birthright to drive cars—nor to spread disease or synthesize DNA. In 2004, I published a white paper advocating for a system of surveillance of synthetic biology, noting technical choke points like phosphoramidite chemicals, enabling devices, and even risk factors in the private lives of trainees. Although individually imperfect, as with all public health innovations, small effects can add up to very high herd immunity—and this is a worthwhile goal toward which to strive.
George Church is a professor at Harvard & MIT, and the co-author of 514 papers, 143 patent publications and the book Regenesis. He co-initiated the BRAIN Initiative and the Human Genome Project.
Không có nhận xét nào:
Đăng nhận xét